Demonstration of the operation of a cold atmospheric pressure plasma jet and its antibacterial effect in microgravity
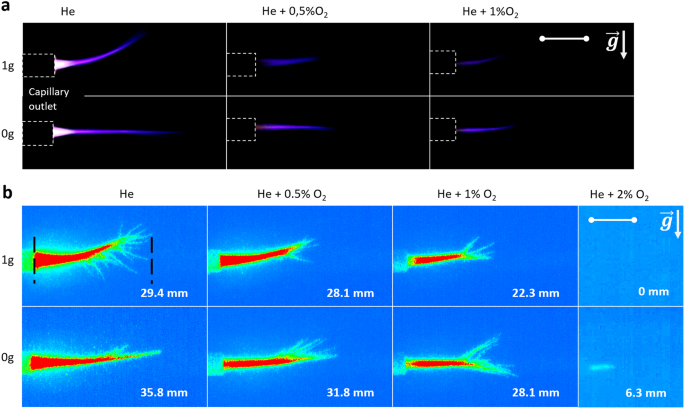
First, we focus on the inhibition mechanisms encountered under our experimental conditions.
For a He gas plasma jet at 7 kV and 20 kHz, under microgravity conditions (0 g), the plasma jet reaches the target at all gap distances and gas admixtures investigated, according to the ICCD records presented in Fig. 1b. The direct contact between plasma and target could explain the clean inactivation area through short-range effects, most likely caused by short-lived reactive species, charged species and UV. The plasma plume has a conical shape at both 0 g and 1 ga. The greater the distance from the reactor, the smaller the section of the plasma plume and hence the contact area with the target surface. This explains the reduced inactivation area at the larger gap (25 mm) compared to the smaller one (15 mm). The same applies to the supply of O2 since this admixture shortens the length of the plume and results in a smaller plasma target cross section and a smaller inhibition area. Inhibition areas obtained under the same operating conditions but at 1 g (Fig. 3d) show similar trends to those at 0 g, but with slightly reduced diameters. This is consistent with the ICCD images showing a shorter plasma plume at 1 g compared to 0 g (Fig. 1b).
To further investigate the assumption that short-lived reactive species play an important role in determining the inhibition range, filtered ICCD images were performed to selectively visualize the location of some of these species. These short-lived species (O*, OH*, NO* in their excited states) are highly reactive and have strong oxidizing and bactericidal activity20,21,22. Since the authors had similar results on bacterial inactivation under terrestrial and microgravity conditions, they assumed that the inactivation mechanisms at 1 g and 0 g are similar. Therefore, all subsequent ICCD analyses were performed under terrestrial conditions. Images were taken during antibacterial experiments using an agar target. However, the signal-to-noise ratio was rather poor and the side wall of the Petri dish prevented easy image acquisition, which limited the clear documentation of the data. Although changing the target affects the plasma characteristics, we checked whether similar emission patterns were observed with an agar plate or a conductive target. Therefore, ICCD-filtered imaging was performed on a flat, grounded metal target to enhance the emission intensity of the species transition under study.
As shown in Fig. 4a, the investigated short-lived RONS, O*, OH* and NO* emissions arise mainly at the plasma-target interface. Note that the OH* filter is not selective enough to exclude any contribution from the second positive nitrogen bands at 315.9 nm, so the corresponding image in Fig. 4a is less informative than that for atomic oxygen and nitric oxide emission patterns. Conversely, the helium emission pattern is much more intense at the tip of the PG capillary, while Fig. 4a also shows a second high intensity zone near the target. The strong population of reactive species in the vicinity of the target results from the impingement and counterpropagation of the plasma jet, as previously discussed for grounded or levitating potential targets.23,24Due to their enormous reactivity, they do not diffuse far from their place of origin, but play an important role in inhibiting bacterial growth.25.
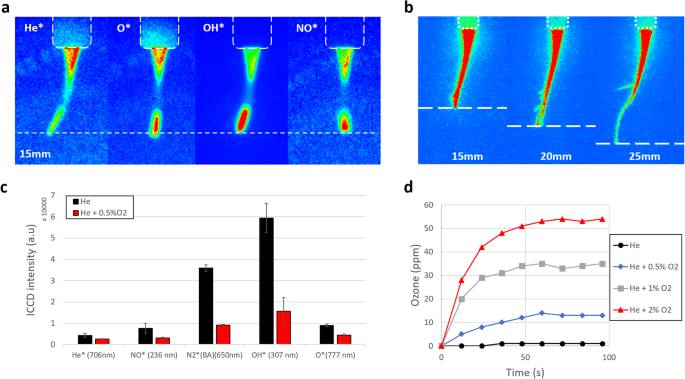
A Filtered ICCD images of a plasma treatment of a grounded target at 15 mm. Contrast and brightness settings are optimally adjusted for each image. B ICCD images of a 7 kV, 20 kHz He plasma treatment of a grounded target compared to different reactor-target distances: 15 mm, 20 mm, 25 mm. Contrast and brightness settings are the same for each image. C ICCD intensities of different transitions on the surface of a grounded target 15 mm away for a He (black) and a He + 0.5% O2 (red) Gas mixture for a plasma at 20 kHz and 7 kV. The values given are mean ± SD of N = 10 repetitions. D Ozone measurement compared to O2 Concentration at 20 kHz and 7 kV. The target is 15 mm away, metallic and grounded. The values given are averages N= 10 repetitions.
As shown in Fig. 4b, larger gap distances lead to smaller plasma-target interfaces, so fewer short-lived RONS are formed and come into contact with the target.
The production of reactive oxygen species (ROS) could be increased by adding a small amount of oxygen. In our case, O2 Addition also leads to a shorter plasma plume and thus to a smaller contact area. To quantify the impact of these two opposing effects of O2 In addition to RONS production, the ICCD uptake intensity near the target was filtered for different O2 The content is documented in Fig. 4c.
Figure 4c shows a decrease in He* production but also in RONS production as the oxygen concentration increases. This indicates that for the same PG configuration, the addition of oxygen to the gas medium quenches the production of O*, NO* (and N2*(BA) which generates NO* by various reactions), OH*, O*, He* radicals at the plasma-plume interface. This is confirmed by previous studies on the quenching effect of oxygen in He plasma.26In a pure He plasma jet, the VUV photons emitted by the He2* promote the ionization of dinitrogen and dioxygen at the plasma jet interface with the ambient air and thus induce RONS production. However, the addition of dioxygen in the plasma consumes the energy of the He electrons in the O2 Arousal and its dissociation27Reduction of O2 and N2 Ionization.
During the addition of more O2 reduces the inhibition area, while at the same time increasing the effective area (Fig. 3b, c). In addition, at 2% the plasma cloud does not reach the target, Fig. 1b, but the effective area diameter reaches its maximum (35 mm). This suggests that other, more extensive mechanisms than those previously proposed are responsible for the observed effective area. We assume that long-lived ROS such as ozone (O3), play an important role. Ozone generated in the plasma reactor can be transported in the gas stream and delivered to the target over considerable distances in sufficient concentration to achieve a bactericidal effect. This assumption is supported by the ozone concentration (Fig. 4d) measured 2 mm from the plasma-target contact point (metal-grounded target) for various He and O concentrations.2 Mixtures: 0%, 0.5%, 1% and 2%.
Figure 4d confirms that the addition of oxygen to the feed gas before the reactor promotes ozone production in the downstream gas stream. As already explained, the addition of O2 in the plasma reactor favored the O2 Dissociation.
$$e+{{\rm{O}}}_{2}\to 2{\rm{O}}+e$$
(1)
Thus, the oxygen atoms could form ozone through a tree body reaction.28
$${\rm{O}}+{{\rm{O}}}_{2}+M\to {{\rm{O}}}_{3}+M$$
(2)
(Mcould be O, O2Ö3He,…)
It is also noteworthy that ozone production increases significantly between 0.5% and 1% oxygen. Interestingly, there is already a notable ozone concentration at 0.5% after 15 s of operation, although no effect region is visible in this case. Therefore, the trends observed for the effect region in Fig. 3b and c could be partially explained by the concentration of O.3 in the initial gas stream. The observed minimum of 0.5% could be a consequence of limited O3 Production (compared to cases with a higher O2 initial content) and a different fluid dynamics compared to the pure He case, which limits the transfer of species to the target surface. Short-lived species, mainly responsible for inactivation areas, are highly reactive and have a strong bactericidal effect. Long-lived species, such as ozone, are less reactive but more far-reaching. They can eventually achieve complete and clean inactivation, but require longer treatment times. However, the time limitation imposed by the parabolic flights prevents the direct test of this hypothesis in 0 g. Further studies on this aspect are currently being carried out in 1 g in the GREMI laboratory. According to Directive 2008/50/EC of the European Parliament and of the Council of 21 May 200829The maximum permissible ozone exposure is 55 ppm for 8 hours per day. The maximum ozone production of the plasma gun is 54 ppm after 96 seconds. Although this is almost the maximum permissible ozone production, a plasma treatment only takes a few minutes. In addition, the measurement was taken close to the point of impact of the plasma jet. Therefore, there is no potential health hazard from ozone exposure from the plasma gun treatment.
Conclude:
-
In this study, the functionality of a cold atmospheric pressure plasma jet in zero gravity was successfully demonstrated.
-
Second, the bactericidal efficacy of PG in 0 g was characterized for different configurations. The inhibitory efficacy of CAPP is higher under parabolic flight conditions than in 1 g.
-
Further investigations have shown that the inactivation mechanisms vary depending on the oxygen concentration in the gas mixture and behave similarly to terrestrial conditions. The more oxygen is added, the smaller the inhibition zone, but the larger the effect zone. This demonstrates the adaptability of CAPP, which optimizes O.2 Levels to precisely control the inactivation process, depending on the application.
-
Further studies are currently underway to investigate the potential effects of cabin negative pressure and new experiments are being conducted to extend the treatment time despite the short time under 0 g conditions.
Overall, this study not only demonstrates the adaptability of CAPP in a weightless environment, but also provides insights into the different inactivation mechanisms and potential applications for decontamination in space. CAPP offers promising opportunities for space research and healthcare in extraterrestrial, confined environments.